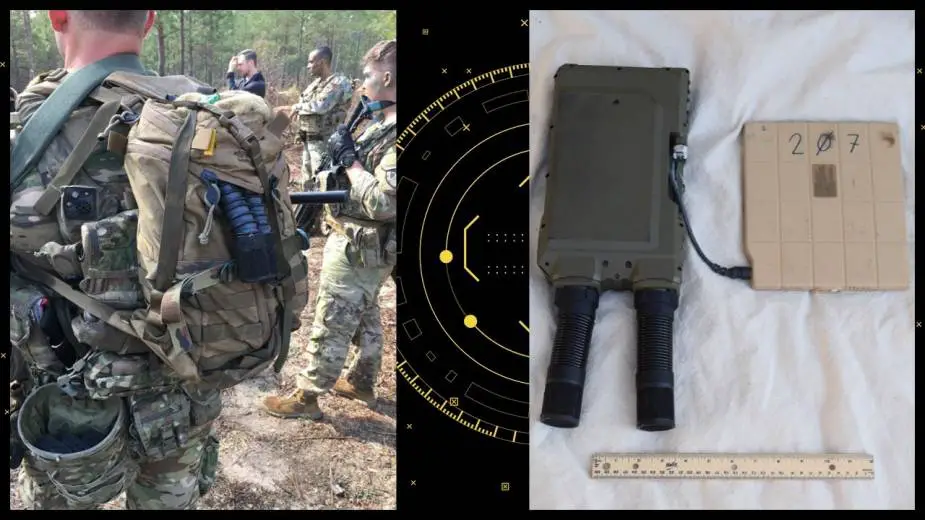
One such innovation uses commonly available, filtered windshield washer fluid — which contains the key ingredient methanol — to recharge Soldiers’ mission-critical electronic devices – such as radios and situational awareness aides – while on the go.
The tool, referred to as the Soldier Wearable Power Generator (SWPG), is a small and mobile fuel cell capable of rendering power through innovative thermal energy technology. Developed in partnership with UltraCell, the SWPG weighs 5 pounds, is designed to be soldier-worn on a backplate or carried in a backpack, and can run off either filtered windshield washer fluid or a commercial methanol/water mix.
When filtered properly, 1 pound of windshield washer fluid can provide enough energy to charge the equivalent of three Conformable Wearable Batteries that weigh 7.8 pounds; the SWPG’s refillable cartridges can be as small as 1 pound or as large as 24 pounds, depending on charging needs.
Beyond charging individual batteries, the SWPG is equipped to directly support tactical, software-based systems such as Nett Warrior. It can also feed battery-charging power scavengers, such as the Universal Battery Charger-Lite and hubs like the Integrated Soldier Power and Data System-Core. While the SWPG provides a 50-watt base load on its own, it can be paired with rechargeable lithium-ion batteries to help fuel hybrid charging systems that offer increased power surges.
The apparatus, which does not get excessively hot or cause ventilation issues for the Soldiers wearing it, seeks to address the Army’s small unit power requirement of providing on-the-move recharging capabilities that extend battery life and minimize the need for frequent battery exchange or reliance on heavy generators.
The Army’s Command, Control, Communications, Computers, Cyber, Intelligence, Surveillance and Reconnaissance (C5ISR) Center leads the service’s applied research and development in energy storage and power generation component technologies. “Army researchers are continually working on solutions to meet Soldiers' anticipated needs during this time of rapid modernization,” said Marnie Bailey, C5ISR Center’s Power Division Chief. “The SWPG is the latest example of using our in-house expertise to enable greater Soldier lethality.”
In addition to being compact, lightweight and more efficient than traditional recharging methods, the SWPG is also significantly quieter than conventional gasoline or diesel-powered generators — an important consideration in combat settings. The reception to the device has been positive, with Soldiers saying that the system does not interfere with their ability to conduct their operations.
US Army prototype uses windshield washer fluid to power soldier electronic devices | weapons defence industry military technology UK | analysis focus army defence military industry army
US Army prototype uses windshield washer fluid to power soldier electronic devices